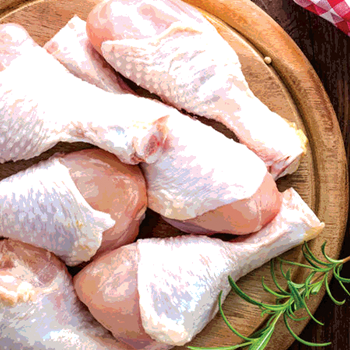
Antibiotic-Free Production and Broiler Chicken Meat Safety
There is a significant amount of research regarding the potential impact of the use of antibiotics in animal feeds on the occurrence of antibiotic resistance. However, there is little information on how the use of antibiotic-free production systems impacts the levels and prevalence of foodborne pathogens on raw meat products.
For many years, poultry feeds have contained subtherapeutic levels of antibiotics, known as antibiotic growth promoters (AGPs), to maximize the growth potential of broiler chickens. With increasing concerns regarding antibiotic resistance, the use of antibiotics in chicken feed has decreased. Antibiotic use in poultry feed can be divided into three general categories: no antibiotics ever (NAE), reduced use, or full spectrum. The NAE category applies to birds that have never been fed any antibiotic during their lifetime, including those that are medically important and those that are not used in human medicine. The reduced-use antibiotic category does not allow the use of medically important antibiotics but does allow the use of antibiotics not used in human medicine. Full spectrum allows any U.S. Food and Drug Administration (FDA)-approved antibiotic to be used in chicken feed. In 2017, 40 percent of broiler chicken feed was provided to chickens raised with NAE.[1] This was increased from 20 percent in 2016. Reduced antibiotic-use feeds increased from 18 to 29 percent and full-spectrum feeds decreased from 28 to 12 percent.
Impacts of NAE Production
Changes in the use of antibiotics in poultry feeds can have major impacts on gut health, specifically with regards to coccidiosis and necrotic enteritis. Coccidiosis is caused by intestinal infection of coccidia protozoan parasites (Eimeria), which are commonly found in fecally contaminated environments, such as chicken growout houses. Infections can cause varying degrees of inflammation, bleeding in the intestine, and damage to epithelial cells. A more severe infection has been associated with increases in Salmonella susceptibility.[2] Since the 1970s, coccidiosis has been controlled through the use of ionophores. Although ionophores are not used in human medicine, they are classified as antibiotics and are thus not used in NAE feeds. Non-antibiotic-classified compounds or coccidia vaccines are now being used in place of ionophores to control infection in NAE birds.[3] Some of the FDA-approved compounds used to treat coccidiosis can include arsenic-based compounds.4 While arsenic-based compounds have been shown to decrease the incidence of Salmonella shedding in chickens,5 there is some concern about potential presence of arsenic in meat.[4] Live coccidial vaccines have been in greater use to manage coccidiosis in the U.S.; however, this may be a challenge in food animals without antibiotics. Breakouts of Eimeria, even at low levels, can cause varying degrees of intestinal damage. It has been suggested that with increased intestinal damage, antibiotic-free birds could be more likely to become susceptible to necrotic enteritis,[3] which occurs typically as a secondary infection of the intestinal epithelium with Clostridium perfringens. C. perfringens is commonly found in healthy animals and proliferates in the intestine upward of 10[6] bacteria per gram of gut content. Although other predisposing conditions can occur, such as issues with diet formulation [large amounts of animal-origin protein or nonstarch polysaccharide (NSP) cereal base without enzymes to break down NSP],[6] changes in the immune status caused by mycotoxins,[7] or viral disease, the most common predisposing factor is coccidiosis.[7] During coccidiosis infection, lesions are formed in the intestine, creating a suitable environment for C. perfringens to proliferate and produce toxins. This secondary infection further damages the intestine, leading to necrosis.[8] Necrotic enteritis induced by coccidiosis can reduce short-term and overall body weight gain compared with healthy birds, limits digestion potential, and increases body-weight variability through market age.[9] The implications of decreased uniformity within a flock due to necrotic enteritis and therefore carcass variability become important for mechanical efficiency during processing. Some work has been done assessing the presence of foodborne pathogens including Salmonella and Campylobacter in the intestines of broilers provided feed with or without antibiotics. A study looking at the presence or absence of bacitracin dimethyl salicylate (BMD) found no differences in cecal Salmonella or ileal Campylobacter prevalence but observed less ileal Salmonella and more cecal Campylobacter at processing age from birds fed with BMD antibiotic added.[10]
Alternative feed ingredients such as prebiotics and probiotics are being used as replacements for antibiotics. Prebiotics are feed ingredients that cannot be digested by the bird but instead selectively stimulate the growth of beneficial bacteria, thus improving bird health. Some of these include nondigestible oligosaccharides (manno-, fructo-, and galacto-oligosaccharides; MOS, FOS, GOS). The beneficial bacteria selected for stimulated growth or activity include Bifidobacteria and Lactobacillus spp., which fall into the category of lactic acid-producing bacteria (LAB). Prebiotics such as MOS can work by blocking binding sites on the intestinal epithelium for pathogens, including Salmonella Typhimurium.[11] Feed additives such as organic acids, inorganic acids, fermentation products, essential oils, and plant extracts can also be added to the diet and/or drinking water to reduce pathogens, including Salmonella.[12] Probiotics, or direct-fed microbials (DFMs), on the other hand, are defined as live microbial feed supplements, which can benefit the bird by improving intestinal microbial balance.[13] Common DFMs include combinations of LABs including Lactobacillus and Bacillus strains with particular lines of species that have been shown to reduce pathogens and potentially improve growth.[14] Probiotics not only affect the bacteria in the intestine but also benefit the bird by regulating the immune response[15,16] and enhancing intestinal integrity. Regulating the bird immune response, including inflammation, is a critical energetic cost to the bird. Currently, the precise modes of action of probiotics are not clear due to the complexity of the bacteria within the gut and a knowledge gap on chicken-microbe relationships. Without understanding the microbial community in the chicken and taking into account the flock-to-flock variability, probiotic administration will remain inconsistent, and breakouts of intestinal disease affecting the final product will continue.
Links between Animal Health and Food Safety
The inclusion of AGPs in feed typically results in the modulation of the intestinal microbial community present, including the suppression of bacterial pathogens,[17] although the mechanisms by which they work are not completely clear. More importantly, AGPs reduce intestinal disease severity, thus decreasing mortality, while improving feed conversion.[18] In the absence or reduction of antibiotic use, there is a pressing need to better understand the role of bacteria in the intestine as they promote health and overall performance. A meta-analysis showed that the need to use AGPs is reduced when nutrition, housing, and hygiene/biosecurity are optimized.[19] Intestinal health has been of increased interest as poultry nutritionists, veterinarians, and producers have attempted to implement new approaches to be better equipped for the changes in live production practices. Although withdrawing AGPs may reduce the risk of creating on-farm antibiotic-resistant bacteria, the use of therapeutic antibiotics increased during the first year after AGPs were banned in Denmark.[19] Early intestinal development is more critical than ever as a growth promotion method with the future discontinued use of antibiotics. During the first week of life, the gut tissues undergo rapid development, increasing the surface area necessary for optimal absorption of nutrients. Intestinal improvements can be seen as increased villus length and lamina propria thickness.[20] If villus growth is impaired during the first 2 weeks of life, this can lead to a permanent limited digestive capacity, reducing growth potential. The early intestinal environment is aerobic, lacks diversity, and contains mostly facultative anaerobic bacteria including enteric bacteria (e.g., Salmonella, Escherichia coli, Klebsiella) as well as Lactobacillus. Over the course of the first few days, bacterial density increases upward to 1011 bacteria per gram of gut content and includes strict anaerobic bacteria (e.g., Clostridium) and different defined communities in each section of the gut.[21] The microbiome of an animal varies in both the density and species as well as in different sections of the intestine. The intestine at an early age is easily susceptible to disease since there is limited colonization resistance, and typically develops as an animal ages.[22] The balance between bird, intestinal bacteria, and the environment is quite delicate, and imbalance can lead to impairments such as an overgrowth of nonspecific intestinal bacteria, leading to enteritis.[23] Intestinal ballooning causing poor gut integrity and malabsorption can lead to wet litter and poor growth.[23] Both poor gut integrity and wet litter can impact processing efficiency and presence of foodborne pathogens on processed
carcasses.
Impact of Intestinal Integrity on Food Safety
Bird health has a significant impact on processing factors directly relating to food safety. For example, broilers with airsacculitis have increased fecal contamination and increased cuts or tears of the digestive tract during processing (Figures 1 and 2).[24] This is thought to be due to variability in bird size due to some birds not being as healthy as others. This size variability becomes a problem because of the high levels of automation necessary during commercial poultry processing. Each piece of equipment is adjusted to operate based on a specific bird size. When birds with smaller or larger body weights enter the equipment, processes such as opening of the body cavity, viscera pack removal, and crop removal become less efficient and can lead to unintended cutting or tearing of viscera. This allows for intestinal contents, which can contain high levels of foodborne pathogens, to come into contact with carcasses on the processing line. In short, bird health impacts flock uniformity, which then leads to potential impacts on food safety.
There are few comparisons between raw products from chickens fed NAE and conventionally raised birds. When retail chicken breasts were sampled for Salmonella, Campylobacter, and coliforms, no differences in prevalence or antibiotic resistances were observed between organic, antibiotic-free, or conventional products (Figure 3).[25] However, in this study, chicken was purchased at a retail market from a variety of sources. In another study where chickens were all processed at the same plant, Salmonella was isolated more frequently from antibiotic-free chicken than conventionally raised birds.[26] A more comprehensive analysis of the impact of NAE programs on the presence of Salmonella and Campylobacter on ready-to-cook poultry meat could potentially be done by assessing regulatory results from both NAE and conventional programs.
To minimize potential negative food safety impacts of antibiotic-free programs on ready-to-cook poultry products, poultry producers are working toward enhancing and maintaining optimum bird health.
Dianna V. Bourassa, Ph.D., is an Assistant Professor/Extension Specialist in poultry processing in the Department of Poultry Science at Auburn University.
Kim M. Wilson, Ph.D., recently completed her doctoral degree at Ohio State University.
References
1. poultryhealthtoday.com/rennier-nae-programs-represented-40-of-us-broiler-feeds-in-2017/.
2. Volkova, VV, et al. 2011. “Associations between Vaccinations against Protozoal and Viral Infections and Salmonella in Broiler Flocks.” Epidemiol Infect 139:206–215.
3. Hofacre, CL, et al. 2018. “An Optimist’s View on Limiting Necrotic Enteritis and Maintaining Broiler Gut Health and Performance in Today’s Marketing, Food Safety, and Regulatory Climate.” Poult Sci 97:1929–1933.
4. Nachman, KE, et al. 2013. “Roxarsone, Inorganic Arsenic, and Other Arsenic Species in Chicken: A U.S.-Based Market Basket Sample.” Env Health Persp 121:818–824.
5. Hofacre, CL, et al. 2007. “Use of Bacitracin and Roxarsone to Reduce Salmonella Heidelberg Shedding Following a Necrotic Enteritis Challenge Model.” J Appl Poult Res 16:275–279.
6. Prescott, JF, et al. 2016. “Experimental Reproduction of Necrotic Enteritis in Chickens: A Review.” Avian Path 45:317–322.
7. Antonissen, G, et al. 2015. “Fumonisins Affect the Intestinal Microbial Homeostasis in Broiler Chickens, Predisposing to Necrotic Enteritis.” Vet Res 46:98.
8. Van Immerseel, F, et al. 2004. “Clostridium perfringens in Poultry: An Emerging Threat for Animal and Public Health.” Avian Path 33:537–549.
9. Wilson, KM, et al. “Comparison of Multiple Methods for Induction of Necrotic Enteritis in Broilers.” J Appl Poul Res in press.
10. Kumar, S, et al. 2018. “Effect of Antibiotic Withdrawal in Feed on Chicken Gut Microbial Dynamics, Immunity, Growth Performance and Prevalence of Foodborne Pathogens.” PLOS ONE 13(2):e0192450.
11. Spring, P, et al. 2000. “The Effects of Dietary Mannaoligosaccharides on Cecal Parameters and the Concentrations of Enteric Bacteria in the Ceca of Salmonella-Challenged Broiler Chicks.” Poult Sci 79:205–211.
12. Cerisuelo, A, et al. 2014. “The Impact of a Specific Blend of Essential Oil Components and Sodium Butyrate in Feed on Growth Performance and Salmonella Counts in Experimentally Challenged Broilers.” Poult Sci 93: 599–606.
13. Fuller, R. 1989. “Probiotics in Man and Animals.” J Appl Bacteriol 66:365–378.
14. Caly, DL, et al. 2015. “Alternatives to Antibiotics to Prevent Necrotic Enteritis in Broiler Chickens: A Microbiologist’s Perspective.” Front Microbiol 6:1336.
15. Lyte, M. 2011. “Probiotics Function Mechanistically as Delivery Vehicles for Neuroactive Compounds: Microbial Endocrinology in the Design and Use of Probiotics.” Bioessays 33:574–581.
16. Vanderpool, C, et al. 2008. “Mechanisms of Probiotic Action: Implications for Therapeutic Applications in Inflammatory Bowel Diseases.” Inflamm Bowel Dis 14:1585–1596.
17. Butaye, P, et al. 2003. “Antimicrobial Growth Promoters Used in Animal Feed: Effects of Less Well Known Antibiotics on Gram-Positive Bacteria.” Clin Microbiol Rev 16:175–188.
18. Dibner, JJ and JD Richards. 2005. “Antibiotic Growth Promoters in Agriculture: History and Mode of Action.” Poult Sci 84:634–643.
19. Jensen, HH and DJ Hayes. 2014. “Impact of Denmark’s Ban on Antimicrobials for Growth Promotion.” Curr Opin Microbiol 19:30–36.
20. Yu, Q, et al. 2012. “Lactobacillus amylophilus D14 Protects Tight Junction from Enteropathogenic Bacteria Damage in Caco-2 Cells.” J Dairy Sci 95:5580–5587.
21. Solis de los Santos, F, et al. 2007. “Gastrointestinal Maturation Is Accelerated in Turkey Poults Supplemented with a Mannan-Oligosaccharide Yeast Extract (Alphamune).” Poult Sci 86:921–930.
22. Kamada, N, et al. 2013. “Control of Pathogens and Pathobionts by the Gut Microbiota.” Nat Immuno 14:685–690.
23. Teirlynck, E, et al. 2011. “Morphometric Evaluation of ‘Dysbacteriosis’ in Broilers.” Avian Path 40:139–144.
24. Russell, SM. 2003. “The Effect of Airsacculitis on Bird Weights, Uniformity, Fecal Contamination, Processing Errors, and Populations of Campylobacter spp. and Escherichia coli.” Poult Sci 82:1326–1331.
25. Mollenkopf, DF, et al. 2014. “Organic or Antibiotic-Free Labeling Does Not Impact the Recovery of Enteric Pathogens and Antimicrobial-Resistant Escherichia coli from Fresh Retail Chicken.” Foodborne Pathog Dis 11:920–929.
26. Park, JH, et al. 2017. “Comparison of the Isolation Rates and Characteristics of Salmonella Isolated from Antibiotic-Free and Conventional Chicken Meat Samples.” Poult Sci 96:2831–2838.